why you can hear gravitational waves when things collide in the universe
- Written by David Blair, Director, WA Node of the ARC Centre of Excellence for Gravitational Wave Discovery, formerly the Australian International Gravitational Research Centre, University of Western Australia
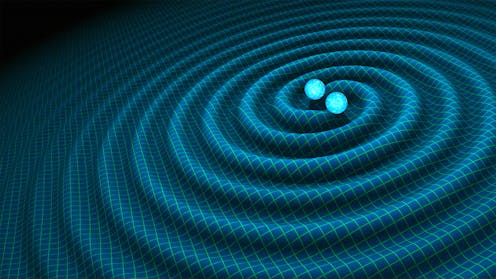
Whenever there’s an announcement of a new discovery of gravitational waves there is usually an accompanying sound, such as this:
The sound of first gravitational waves detected.We have only detected half a dozen signals so far. The first five are black holes whose chirp signal is extremely brief. The last one is the sound of a pair of neutron stars spiralling together. This signal lasted more than a minute.
Listen for the chirp.With good earphones (and good ears) you may be able to hear the lower frequencies but the final chirp is unmistakable. A friend commented that the sound is like the call of the Australian whip bird.
Read more: At last, we've found gravitational waves from a collapsing pair of neutron stars
So what is this sound? Are we really hearing gravitational waves?
To answer that it helps to think about other devices that detect waves, such as seismometers.
Listening for an earthquake
Today a worldwide network of seismometers listens to the Earth as it continuously vibrates. You can hear some examples of earthquakes online at the US Geological Survey (USGS).
Behind the idea of the seismometer is inertia: the linking of mass to space. You feel inertia when you push a car. The more massive the car, the more slowly it speeds up. Inertia resists changes in the motion of matter through space.
In a seismometer a large, delicately suspended mass is freed from most forces, so its inertia links it to space. In an earthquake the ground suddenly shakes. If you feel an earthquake you are feeling sudden changes in your motion through space.
In the seismometer the suspended mass stays still because of its inertia, but the frame of the seismometer follows the shaking Earth. From inside the seismometer it seems as if the mass has suddenly moved.
Can you hear it?
Sound is a wave of vibration that we normally hear passing through the air, but we can also hear under water and through solids. Seismic waves are waves of vibration passing through the solid Earth and this is what is picked up by modern seismometers.
If you were to insist that sound must be audible to us humans, then we can’t actually hear most earthquakes. This is because the vibration frequency is usually lower than the frequency threshold of human hearing.
But record it and speed up the playback and you can hear symphonies of seismic sounds like those you can listen to here at the USGS.
Gravitational waves are waves of stretching and shrinking space. Like seismometers, gravitational wave detectors use the principle of inertia, but in this case the action is reversed. Space expands and shrinks and inertia causes suspended masses to follow.
Motions of space cannot be detected at a single location, for the same reason you cannot measure the stretching of an elastic band at a single point. But a pair of separated masses will become closer together if space shrinks, and further apart if space expands. Inertia makes the masses follow space, but neither mass feels a force.
Similarly, the expansion of space in the universe, that proved the Big Bang theory, causes all the galaxies to be moving apart without any force driving them. But gravitational waves are different in one respect: they simultaneously stretch in one direction and shrink the perpendicular direction.
In essence, gravitational waves vibrate the spacing between masses. The effects are tiny, so each mass must be exquisitely suspended so that it is not affected by the vibrations of the ground, and held in vacuum so that sounds in the air do not affect them.
The big breakthrough
It took 50 years of technology development before we had enough sensitivity to measure these waves. The masses we use are 40kg mirrors and lasers are used to measure their spacing.
Gravitational waves cause he mirrors to move.Albert Einstein’s theory of general relativity tells us that space and time are elastic. Spacetime is an elastic medium that is actually a billion billion billion times stiffer than steel.
We are constantly moving freely through this elastic medium, but we have to apply forces using muscles or tires or rocket engines to allow us to overcome the principle of inertia, thereby changing our motion through space as we have to when we move around relative to other objects.
If a pair of black holes orbit each other they make enormous deformations in the shape of space around them, and because of space’s elasticity, this creates ripples of space that spread out like ripples on a pond.
Watch the ripples until the black holes collide.When the ripples pass pairs of masses, the rippling distortions of space cause the spacing between the masses to fluctuate in unison. But as an observer you simply see that the spacing between the masses is vibrating. It looks like a sound and it sounds like a sound! The only catch is that it needs to be amplified a billion times to be audible.
The first signal itself was detected as a vibration of the distance between mirrors four kilometres apart. They changed their spacing by about a billionth of the diameter of an atom.
Sound carries a wealth of information. Think of the vast number of characteristic sounds we all know, from a dripping tap to a breaking window, from a bird call to a kettle drum. The sound describes the system. For gravitational waves we are able to make precise predictions of the differences in gravitational wave sounds from many systems.
The whoops and chirps we have heard are all different, because the sounds depend on the masses of the black holes, how fast they are spinning and how they are oriented relative to the Earth. Neutron stars are much less massive than the black holes we have heard so far, and this causes the pitch of the signal to rise much more slowly.
All the gravitational waves we have heard so far are in the audio frequency range for the human ear: we really could hear them if our ears were sensitive enough, but real signals are just too quiet.
In the future gravitational wave detectors in space will be able to listen to gravitational waves at much lower frequencies. These, like earthquakes, will need to be sped up for us to hear them.
Gravitational wave detectors are supersensitive microphones for the sounds of space. They amplify the sound, and we get to hear the sound of gravitational waves that have travelled to us from far away in the universe. The universe speaks to us.
Read more: Gravitational waves discovered: the universe has spoken
A better detector
From the recent discoveries we can make predictions of what we might hear as we improve the detectors. As sensitivity improves you increase the range of detection and this increases the volume of the universe you can listen to by the cube of the improvement factor.
Every two times improvement gives eight times as many signals. In the next few years the three detectors in the world: two LIGO detectors in the US and the Virgo detector in Europe should be detecting hundreds of black holes and neutron stars colliding every year. Two more detectors are under construction in Japan and India.
More detectors helps to pinpoint where signals come from, but the biggest pay off comes from increasing sensitivity. Just four-fold further improvement would expand our horizon to encompass about half of the visible universe while a ten-fold improvement would give us the whole universe. Detectors with this capability have been suggested for Australia, China, Europe and the US.
The legacy of Einstein, the recent Nobel Prize winners and the huge international team of gravitational wave physicists that made the first discoveries, will be the ability to listen to the whole universe, and to hear it running down as black holes form and grow.
Gravitational waves are truly a new sense for humanity. We are no longer deaf to the sounds of space. We can be pretty certain that our new-found sense will bring with it surprises and unforeseen revelations, as well as remarkable new technologies.
Authors: David Blair, Director, WA Node of the ARC Centre of Excellence for Gravitational Wave Discovery, formerly the Australian International Gravitational Research Centre, University of Western Australia